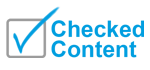
Darmstadtium
Background Information
SOS Children has tried to make Wikipedia content more accessible by this schools selection. SOS Child sponsorship is cool!
Darmstadtium | |||||||||||||||||||||||||||||||||||||
---|---|---|---|---|---|---|---|---|---|---|---|---|---|---|---|---|---|---|---|---|---|---|---|---|---|---|---|---|---|---|---|---|---|---|---|---|---|
110Ds
|
|||||||||||||||||||||||||||||||||||||
|
|||||||||||||||||||||||||||||||||||||
Appearance | |||||||||||||||||||||||||||||||||||||
unknown | |||||||||||||||||||||||||||||||||||||
General properties | |||||||||||||||||||||||||||||||||||||
Name, symbol, number | darmstadtium, Ds, 110 | ||||||||||||||||||||||||||||||||||||
Pronunciation | / d ɑr m ˈ ʃ t ɑː t i ə m / darm-SHTAHT-ee-əm |
||||||||||||||||||||||||||||||||||||
Metallic category | unknown but probably a transition metal |
||||||||||||||||||||||||||||||||||||
Group, period, block | 10, 7, d | ||||||||||||||||||||||||||||||||||||
Standard atomic weight | [281] | ||||||||||||||||||||||||||||||||||||
Electron configuration | [Rn] 5f14 6d8 7s2 (predicted) 2, 8, 18, 32, 32, 16, 2 (predicted) |
||||||||||||||||||||||||||||||||||||
History | |||||||||||||||||||||||||||||||||||||
Discovery | Gesellschaft für Schwerionenforschung (1994) | ||||||||||||||||||||||||||||||||||||
Physical properties | |||||||||||||||||||||||||||||||||||||
Phase | solid (predicted) | ||||||||||||||||||||||||||||||||||||
Density (near r.t.) | 34.8 (predicted) g·cm−3 | ||||||||||||||||||||||||||||||||||||
Atomic properties | |||||||||||||||||||||||||||||||||||||
Oxidation states | 8, 6, 4, 2, 0 (predicted) | ||||||||||||||||||||||||||||||||||||
Ionization energies ( more) |
1st: 955.2 (estimated) kJ·mol−1 | ||||||||||||||||||||||||||||||||||||
2nd: 1891.1 (estimated) kJ·mol−1 | |||||||||||||||||||||||||||||||||||||
3rd: 3029.6 (estimated) kJ·mol−1 | |||||||||||||||||||||||||||||||||||||
Atomic radius | 118 (estimated) pm | ||||||||||||||||||||||||||||||||||||
Covalent radius | 128 (estimated) pm | ||||||||||||||||||||||||||||||||||||
Miscellanea | |||||||||||||||||||||||||||||||||||||
CAS registry number | 54083-77-1 | ||||||||||||||||||||||||||||||||||||
Most stable isotopes | |||||||||||||||||||||||||||||||||||||
Main article: Isotopes of darmstadtium | |||||||||||||||||||||||||||||||||||||
|
|||||||||||||||||||||||||||||||||||||
Darmstadtium is a chemical element with the symbol Ds and atomic number 110. It is an extremely radioactive synthetic element (an element that can be created in a laboratory but is not found in nature). The most stable known isotope, darmstadtium-281, has a half-life of approximately 11 seconds, but it is possible that this darmstadtium isotope may have an isomer with a longer half-life, 3.7 minutes. Darmstadtium was first created in 1994 by the GSI Helmholtz Centre for Heavy Ion Research near Darmstadt, Germany. It was named after the city of Darmstadt, where it was discovered.
In the periodic table, it is a d-block transactinide element. It is a member of the 7th period and is placed in the group 10 elements, although no chemical experiments have yet been carried out to confirm that it behaves as the heavier homologue to platinum in group 10. Darmstadtium is calculated to have similar properties to its lighter homologues, nickel, palladium, and platinum.
History
Discovery
Darmstadtium was first created on November 9, 1994, at the Institute for Heavy Ion Research (Gesellschaft für Schwerionenforschung) in Darmstadt, Germany, by Peter Armbruster and Gottfried Münzenberg, under the direction of Sigurd Hofmann. The team bombarded a lead-208 target with accelerated nuclei of nickel-62 and detected a single atom of the isotope darmstadtium-269:
- 208
82Pb + 62
28Ni → 269
110Ds + 1
0n
In the same series of experiments, the same team also carried out the reaction using heavier nickel-64 ions. During two runs, 9 atoms of 271Ds were convincingly detected by correlation with known daughter decay properties:
- 208
82Pb + 64
28Ni → 271
110Ds + 1
0n
The IUPAC/IUPAP Joint Working Party (JWP) recognised the GSI team as discoverers in their 2001 report.
Naming
The name darmstadtium (Ds) was suggested by the GSI team in honour of the city of Darmstadt, where the element was discovered. The GSI team originally also considered naming the element wixhausium, after the suburb of Darmstadt known as Wixhausen where the element was discovered, but eventually decided on darmstadtium. The new name was officially recommended by IUPAC on August 16, 2003.
Nucleosynthesis
Super-heavy elements such as darmstadtium are produced by bombarding lighter elements in particle accelerators that induce fusion reactions. Whereas most of the isotopes of darmstadtium can be synthesized directly this way, some heavier ones have only been observed as decay products of elements with higher atomic numbers.
Depending on the energies involved, the former are separated into "hot" and "cold". In hot fusion reactions, very light, high-energy projectiles are accelerated toward very heavy targets (actinides), giving rise to compound nuclei at high excitation energy (~40–50 MeV) that may either fission or evaporate several (3 to 5) neutrons. In cold fusion reactions, the produced fused nuclei have a relatively low excitation energy (~10–20 MeV), which decreases the probability that these products will undergo fission reactions. As the fused nuclei cool to the ground state, they require emission of only one or two neutrons, and thus, allows for the generation of more neutron-rich products. The latter is a distinct concept from that of where nuclear fusion claimed to be achieved at room temperature conditions (see cold fusion).
Cold fusion
Before the first successful synthesis of darmstadtium in 1994 by the GSI team, scientists at GSI also tried to synthesize darmstadtium by bombarding lead-208 with nickel-64 in 1986. No darmstadtium atoms were identified. After an upgrade of their facilities, the team at GSI successfully detected 9 atoms of 271Ds in two runs of their discovery experiment in 1994. This reaction was successfully repeated in 2000 by GSI (4 atoms), in 2000 and 2004 by the Lawrence Berkeley National Laboratory (LBNL) (9 atoms in total) and in 2002 by RIKEN (14 atoms). The GSI team studied the analogous reaction with nickel-62 instead of nickel-64 in 1994 as part of their discovery experiment. Three atoms of 269Ds were detected. A fourth decay chain was measured but was subsequently retracted.
In addition to the official discovery reactions, in October–November 2000, the team at GSI also studied the analogous reaction using a lead-207 target in order to synthesize the new isotope 270Ds. They succeeded in synthesising 8 atoms of 270Ds, relating to a ground state isomer, 270Ds, and a high- spin metastable state, 270mDs.
In 1986, a team at the Joint Institute for Nuclear Research (JINR) in Dubna, Russia, studied the reaction:
- 209
83Bi + 59
27Co → 267
110Ds + 1
0n
They were unable to detect any darmstadtium atoms. In 1995, the team at LBNL reported that they had succeeded in detecting a single atom of 267Ds using this reaction. However, several decays were not measured and further research is required to confirm this discovery.
Hot fusion
In September 1994, the team at Dubna detected a single atom of 273Ds by bombarding a plutonium-244 target with accelerated sulfur-34 nuclei.
Experiments have been performed in 2004 at the Flerov Laboratory of Nuclear Reactions in Dubna studying the fission characteristics of the compound nucleus 280Ds, produced through the nuclear reaction:
- 232
90Th + 48
20Ca → 280
110Ds* → fission
The result revealed how compound nuclei such as this fission predominantly by expelling magic and doubly-magic nuclei such as 132Sn (Z=50, N=82). No darmstadtium atoms were obtained. A compound nucleus is a loose combination of nucleons that have not arranged themselves into nuclear shells yet. It has no internal structure and is held together only by the collision forces between the target and projectile nuclei. It is estimated that it requires around 10−14 s for the nucleons to arrange themselves into nuclear shells, at which point the compound nucleus becomes an nuclide, and this number is used by IUPAC as the minimum half-life a claimed isotope must have to potentially be recognised as being discovered. Thus, the isotope 280Ds currently remains unknown.
As decay product
Evaporation residue | Observed darmstadtium isotope |
---|---|
277Cn | 273Ds |
285Fl, 281Cn | 277Ds |
291Lv, 287Fl, 283Cn | 279Ds |
293Lv, 289Fl, 285Cn | 281Ds |
Darmstadtium has been observed as decay products of copernicium. Copernicium currently has six known isotopes, four of which have been shown to undergo alpha decays to become darmstadtium nuclei, with mass numbers between 273 and 281. Darmstadtium isotopes with mass numbers 277, 279 and 281 to date have only been produced by copernicium nuclei decay. Parent copernicium nuclei can be themselves decay products of flerovium or livermorium. To date, no other elements have been known to decay to darmstadtium. For example, in 2004, the Dubna team ( JINR) identified darmstadtium-281 as a product in the decay of livermorium via an alpha decay sequence:
- 293
116Lv → 289
114Fl + 4
2He - 289
114Fl → 285
112Cn + 4
2He - 285
112Cn → 281
110Ds + 4
2He
Isotopes
Isotope |
Half-life |
Decay mode |
Discovery year |
Reaction |
---|---|---|---|---|
267Ds ? | 2.8 μs | α | 1994 | 209Bi(59Co,n) |
268Ds | 100? μs | α ? | unknown | — |
269Ds | 179 μs | α | 1994 | 208Pb(62Ni,n) |
270Ds | 100 μs | α, SF | 2000 | 207Pb(64Ni,n) |
270mDs | 6.0 ms | α, IT | 2000 | 207Pb(64Ni,n) |
271Ds | 1.63 ms | α | 1994 | 208Pb(64Ni,n) |
271mDs | 69 ms | α | 1994 | 208Pb(64Ni,n) |
272Ds | 1? s | SF ? | unknown | — |
273Ds | 170 μs | α | 1996 | 244Pu(34S,5n) |
274Ds | 2? s | α, SF ? | unknown | — |
275Ds | 2? s | α ? | unknown | — |
276Ds | 5? s | α ? | unknown | — |
277Ds | 5.7 ms | α | 2010 | 285Fl(—,2α) |
278Ds | 10? s | α, SF ? | unknown | — |
279Ds | 0.18 s | α, SF | 2002 | 291Lv(—,3α) |
280Ds | 11? s | SF ? | unknown | — |
281Ds | 11 s | SF | 1999 | 289Fl(—,2α) |
281mDs ? | ~3.7 min | α | 1999 | 289Fl(—,2α) |
Darmstadtium has no stable or naturally-occurring isotopes. Several radioactive isotopes have been synthesized in the laboratory, either by fusing two atoms or by observing the decay of heavier elements. Eight different isotopes of darmstadtium have been reported with atomic masses 267, 269–271, 273, 277, 279, and 281, although darmstadtium-267 is unconfirmed. Three darmstadtium isotopes, darmstadtium-270, darmstadtium-271, and darmstadtium-281, have known metastable states (although that of darmstadtium-281 is unconfirmed). Most of these decay predominantly through alpha decay, but some undergo spontaneous fission.
Stability and half-lives
All darmstadtium isotopes are extremely unstable and radioactive; in general, the heavier isotopes are more stable than the lighter. The most stable known darmstadtium isotope, 281Ds, is also the heaviest known darmstadtium isotope; it has a half-life of 11 seconds, although a metastable state, 281mDs, has been reported to have a longer half-life of about 3.7 minutes. The isotope 279Ds has a half-life of 0.18 seconds respectively. The remaining six isotopes and two metastable states have half-lives between 1 microsecond and 70 milliseconds. Some unknown isotopes in this region, such as 272Ds, 274–276Ds, and 280Ds, are predicted to also have rather long half-lives of a few seconds. Before its discovery, 277Ds was predicted to also have a long half-life of around 5 seconds; however, it has since been found to have a very short half-life of only 5.7 milliseconds.
The undiscovered isotope 284Ds has been predicted to be the most stable towards beta decay; however, no known darmstadtium isotope has been observed to undergo beta decay. Theoretical calculation in a quantum tunneling model reproduces the experimental alpha decay half-life data for the known darmstadtium isotopes. It also predicts that the undiscovered isotope 294Ds, which has a magic number of neutrons (184), would have an alpha decay half-life on the order of 311 years.
Nuclear isomerism
- 281Ds
The production of 281Ds by the decay of 289Fl or 293Lv has produced two very different decay modes. The most common and readily confirmed mode is spontaneous fission with a half-life of 11 s. A much rarer and as yet unconfirmed mode is alpha decay by emission of an alpha particle with energy 8.77 MeV with an observed half-life of around 3.7 min. This decay is associated with a unique decay pathway from the parent nuclides and must be assigned to an isomeric level. The half-life suggests that it must be assigned to an isomeric state but further research is required to confirm these reports.
- 271Ds
Decay data from the direct synthesis of 271Ds clearly indicates the presence of two nuclear isomers. The first emits alpha particles with energies 10.74 and 10.69 MeV and has a half-life of 1.63 ms. The other only emits alpha paricles with an energy of 10.71 MeV and has a half-life of 69 ms. The first has been assigned to the ground state and the latter to an isomeric level. It has been suggested that the closeness of the alpha decay energies indicates that the isomeric level may decay primarily by delayed isomeric transition to the ground state, resulting in an identical measured alpha energy and a combined half-life for the two processes.
- 270Ds
The direct production of 270Ds has clearly identified two nuclear isomers. The ground state decays by alpha emission into the ground state of 266Hs by emitting an alpha particle with energy 11.03 MeV and has a half-life of 0.10 ms. The metastable state decays by alpha emission, emitting alpha particles with energies of 12.15, 11.15, and 10.95 MeV, and has a half-life of 6 ms. When the metastable state emits an alpha particle of energy 12.15 MeV, it decays into the ground state of 266Hs, indicating that it has 1.12 MeV of excess energy.
Predicted properties
Chemical
Darmstadtium is the eighth member of the 6d series of transition metals. Since copernicium (element 112) has been shown to be a transition metal, it is expected that all the elements from 104 to 112 would form a fourth transition metal series, with darmstadtium as part of the platinum group metals. Calculations on its ionization potentials and atomic and ionic radii are similar to that of its lighter homologue platinum, thus implying that darmstadtium's basic properties will resemble those of the other group 10 elements, nickel, palladium, and platinum.
Prediction of the probable chemical properties of darmstadtium has not received much attention recently. Darmstadtium is expected to be a noble metal. Based on the most stable oxidation states of the lighter group 10 elements, the most stable oxidation states of darmstadtium are predicted to be the +6, +4, and +2 states; however, the neutral state is predicted to be the most stable in aqueous solutions. In comparison, only platinum is known to show the maximum oxidation state in the group, +6, while the most stable states are +4 and +2 for both nickel and palladium. It is further expected that the maximum oxidation states of elements from bohrium (element 107) to darmstadtium (element 110) may be stable in the gas phase but not in aqueous solution. Darmstadtium hexafluoride (DsF6) is predicted to have very similar properties to its lighter homologue platinum hexafluoride (PtF6), having very similar electronic structures and ionization potentials.
Physical and atomic
Darmstadtium is expected to be a solid under normal conditions. It should be a very heavy metal with a density of around 34.8 g/cm3. In comparison, the densest known element that has had its density measured, osmium, has a density of only 22.61 g/cm3. This results from darmstadtium's high atomic weight, the lanthanide and actinide contractions, and relativistic effects, although production of enough darmstadtium to measure this quantity would be impractical, and the sample would quickly decay.
The outer electron configuration of darmstadtium is calculated to be 6d87s2, which obeys the Aufbau principle and does not follow platinum's outer electron configuration of 5d96s1. This is due to the relativistic stabilization of the 7s2 electron pair over the whole seventh period, so that none of the elements from 104 to 112 have electron configurations violating the Aufbau principle. The atomic radius of darmstadtium is expected to be around 118 pm.
Experimental chemistry
Unambiguous determination of the chemical characteristics of darmstadtium has yet to have been established due to the short half-lives of darmstadtium isotopes and a limited number of likely volatile compounds that could be studied on a very small scale. One of the few darmstadtium compounds that are likely to be sufficiently volatile is darmstadtium hexafluoride (DsF6), as its lighter homologue platinum hexafluoride (PtF6) is volatile above 60 ºC and therefore the analogous compound of darmstadtium might also be sufficiently volatile; a volatile octafluoride (DsF8) might also be possible. For chemical studies to be carried out on a transactinide, at least four atoms must be produced, the half-life of the isotope used must be at least 1 second, and the rate of production must be at least one atom per week. Even though the half-life of 281Ds, the most stable confirmed darmstadtium isotope, is 11 seconds, long enough to perform chemical studies, another obstacle is the need to increase the rate of production of darmstadtium isotopes and allow experiments to carry on for weeks or months so that statistically significant results can be obtained. Separation and detection must be carried out continuously to separate out the darmstadtium isotopes and automated systems can then experiment on the gas-phase and solution chemistry of darmstadtium as the yields for heavier elements are predicted to be smaller than those for lighter elements; some of the separation techniques used for bohrium and hassium could be reused. However, the experimental chemistry of darmstadtium has not received as much attention as that of the heavier elements copernicium and flerovium.
The more neutron-rich darmstadtium isotopes are the most stable and are thus more promising for chemical studies; however, they can only be produced indirectly from the alpha decay of heavier elements, and indirect synthesis methods are not favourable for chemical studies. The more neutron-rich isotopes 276Ds and 277Ds might be produced directly in the reaction between thorium-232 and calcium-48, but the yield is expected to be low. Furthermore, this reaction has already been tested without success, and more recent experiments that have successfully synthesized 277Ds using indirect methods show that it has a short half-life of 5.7 ms, not long enough to perform chemical studies.